Disclaimer & Copyright Notices; Optimized for the MS Edge
Phosphorus species
Soil & Water Conservation Society of Metro Halifax (SWCSMH)
Updated: June 04, 2017
Acknowledgements to Prof. T. L. Carosella for the picture
Contents:
Phosphorus, The Morning Star
(Vallentyne, 1974)
In 1669, Hennig Brand of Hamburg
brewed a concoction that yielded a mysterious product; one that, unlike
any other substance known at the time, glowed in the dark by its own
power and ignited spontaneously when exposed to air. He called it
phosphorus, meaning light-bearing, from the name given by ancient Greek
and Roman astronomers to the planet Venus when it appeared in the sky
as the morning star.
Of several hundred phosphorus
compounds isolated from living cells, three deserve particular mention:
DNA (deoxyribonucleic acid) and RNA (ribonucleic acid) are general
classes of polymers, and ATP (adenosine triphosphate) is a small
molecule that drives energy-requiring synthetic reactions in cells.
Phosphates enter into the
economy of man in many different ways. Their principal uses are: in
fertilizers, in detergents, in animal feeds, and the remaining
distributed among a variety of applications such as leavening agents in
baking powders and cake mixes; anticorrosion agents for car bodies;
fireproofing materials; chemical agents in some types of water
softeners; smoke bombs and incendiaries; insecticides; rodent poisons
and lethal nerve gases; and as additives to gasoline, "muds" used in
oil drilling operations, bottled "pops" and tonics, toothpastes, dental
cements, matches, and plasticizers.
Note how the uses range from
life-promoting fertilizers to deadly poisons intended for rodents,
insects, and man. One drop of sarin, a lethal nerve gas containing
phosphorus, if not immediately washed from the skin, can kill a man as
rapidly as a bullet through the brain.
As little as 0.6 mg (0.00002
oz in weight) of elemental phosphorus, an amount equivalent to a few
grains of salt, can induce hepatitis in man. A dose of 100 mg (0.003
oz) results in death.
Pathways to a water body
Phosphorus may enter a water body through
the inflows, precipitation, dry fallout and from the sediments, and it
may be removed by sedimentation and through the outflow. Nitrogen has a
more complex pathway. In addition to the inputs and outputs described
for phosphorus, nitrogen can enter and leave a water body in the form
of free nitrogen gas (N2-) through atmospheric exchange.
Carbon has been shown to diffuse into the water column at rates
sufficient to meet the needs of photosynthesizing cells. Phosphorus, on
the other hand, cycles between living and nonliving particulate forms
and the dissolved form. The different pathways of phosphorus, nitrogen
and carbon in lake metabolism make phosphorus the obvious choice for
eutrophication control. A certain reduction of phosphorus input will
generally result in a greater reduction in algal biomass compared with
the same reduction of nitrogen. Furthermore, the reduction of nitrogen
input without a proportional reduction in phosphorus, creates low N/P
ratio which favors nitrogen fixing nuisance algae, without any
reduction in algal biomass.
Diagram showing relative importance of phosphorus availability at the molecular level and according to sources:
(Vollenweider, 1979)
![[Img-tp1.jpg]](PIC/tp1.jpg)
Forms of Phosphorus
Total Phosphorus and not other phosphorus
species, is considered the key variable for practical rather than
theoretical reasons. Total phosphorus includes some or all of the
following fractions: crystalline, occluded, absorbed, particulate
organic, soluble organic and soluble inorganic phosphorus. Out of these
fractions, the three biologically available phosphorus (BAP) fractions
listed in order of decreasing availability are soluble reactive
phosphorus (SRP, a mixture of dissolved inorganic and organic species
measured by the method of Murphy and Riley, 1962),
soluble unreactive phosphorus (SURP, some include dissolved phosphorus
fed by persulfate oxidation, and is available for phytoplankton by
enzimatic hydraulisation which frees organically bound fractions), and
"labile" phosphorus (associated with soil particles and dissolves into
an aqueous solution as determined by the sorption isotherm method of
Tayler and Kunishi, 1971). However, the term biologically available
phosphorus (BAP) still remains somewhat vague because it describes a
mixture of phosphorus fractions of different availability.
Forms of Phosphorus in water:
(Haygarth, 1997)
- Forms of total P (TP) in water are determined by analytical methods based around:
- Whether the P is in a dissolved or particulate form. This is most often differentiated by a 0.45 µm membrane filtration.
- Whether or not the P is molybdate (Mo) reactive, according to the Mo blue reaction (Murphy and Riley, 1962).
As a general rule, Mo reactive P (RP) is considered to be inorganic and
unreactive P (UP) is thought to represent organic P. RP is free and
readily mobile. UP is less labile and can only be determined as the
difference between total P and RP. Total P is determined by an
appropriate means of digestion (Rowland and Haygarth, 1997) which hydrolyses forms of P which would be otherwise occluded from Mo reaction.
- Thus, by applying the combination of Mo reaction, digestion and filtration it is possible to define nine forms of P in water.
Analytical methods for P determination:
![[Img-tp2.jpg]](PIC/tp2.jpg)
Internal Loading of Phosphorus in lakes
Where suitable conditions develop at the
water sediment interface, substances contained in the sediments,
including nutrients, are released into the water column. Below
compensation depth (in the tropholytic zone), net oxygen consumption
occurs in a eutrophic lake. As alternate TEAs (terminal electron
acceptors) are consumed, Eh (environmental redox potential) decreases.
Eh tends to decrease with greater depth in the water column and in
sediments. Once the Eh of the ferric-ferrous iron couple is reached (@
approx. 120 mv, Kortmann & Rich 1994),
both soluble ferrous iron and soluble phosphate accumulate. If Eh
continues to decrease, sulfate is reduced to sulfide (@ <-75 mv, Kortmann & Rich 1994),
which can remove iron and permanently reduce phosphate binding
capacity, by interacting readily with ferrous iron to produce ferrous
sulfide (FeS). If FeS precipitates to form pyrite (FeS2),
ferrous iron is no longer susceptible to oxidation to ferric iron with
the return of aerobic conditions. Bacterial reduction of ferric
hydroxy-phosphate complexes results in large amounts of soluble
inorganic phosphorus and ferrous iron diffusing across the
sediment-water interface causing internal loading. The relationships
among sulfur, iron, and phosphorus binding capacity raises questions
about potential impacts from increased sulfate loading by algicide
applications (copper sulfate), alum treatments (aluminum sulfate), and
acid rain (sulfuric acid). Ferrous iron is reoxidized when it
encounters oxygen (from the trophogenic zone) to insoluble ferric iron
which binds with orthophosphate. Accumulated hypolimnetic phosphorus
can be transported to the trophogenic zone by eddy transport or wind
mixing episodes, and can have dramatic effects on autochthonous
production.
It is now widely recognized
that the mixing processes (principally induced by wind force, sometimes
even by several high power motorboats) which occur between sediments
and the overlying water play an important role in the overall
phosphorus release from the sediments under both oxic and anoxic
conditions. This seems to confirm that internal loading of phosphorus
is a more serious threat in shallow lakes than in deep lakes, where in
the latter case, the substances released are prevented from entering
the epilimnium during periods of thermal stratification. Experience
gained in various lake restoration schemes suggests that the history of
accelerated eutrophication, that is, the length of time the lake has
been eutrophied, has an important bearing on lake behavior with respect
to internal loading and phosphorus retention in the sediments.
Sediments remain oligotrophic and only become gradually eutrophic, long
after the water mass becomes highly eutrophic (Schindler et al).
Conversely, the highly eutrophic sediment would remain eutrophic long
after the external load is reduced and would thus delay the recovery of
the lake. In some shallow highly eutrophied lakes with a long history
with eutrophication (Ryding and Forsberg), 22 to 400% of the external
phosphorus load was released from the sediments after reduction of the
external load.
Phosphorus is also released from lake sediments to well aerated water. Jensen and Andersen (1992)
have shown that Fe-bound P, when present in significant proportions in
the sediment, may be a major source for internal P loading in shallow,
eutrophic lakes, just as it may be in deeper, stratified lakes. Holdren
and Armstrong (1980) per Fricker (1981)
quoted literature values of sediment phosphorus release rates from
several lakes in the United States for aerobic (0 to 13 mg P m-2 day-1) and anaerobic conditions (0 to 50 [max. 150] mg P m-2 day-1).
Phosphorus mobilization and transport:
Two different mechanisms have to occur
simultaneously or within a short space of time. Firstly, P bound to
particles or aggregates in the sediment must be mobilized by being
transferred to the pool of dissolved P (primarily phosphate) in the
pore water. Secondly, processes which transport the dissolved
phosphorus to the lake water must function. Important mobilization
processes are desorption, dissolution, ligand exchange mechanisms, and
enzymatic hydrolysis. These processes are affected by a number of
environmental factors, of which redox potential, pH and temperature are
the most important. Essential transport mechanisms are diffusion,
wind-induced turbulence, bioturbation, and gas convection.
Redox-controlled dissolution and diffusion are considered as the
dominant mechanisms for P release from stagnant hypolimnetic bottom
areas. All the mobilization and transport processes can theoretically
contribute to the overall P release from sediments in shallow lakes. At
high temperatures microanaerobic zones are formed very rapidly, and
redox-controlled liberation of phosphate can occur to well-aerated
water. Wind-induced turbulences often have a dominating role among the
transport processes.
Effects of important environmental factors on P mobilization (Boström et al, 1982)
Environmental parameter | Effect on phosphorus mobilization |
Redox potential | Fe-bound P is released at potentials below 200 mV when Fe(III) is reduced to Fe(II) |
pH | An increase in pH decreases the P-binding capacity
of Fe and Al compounds, primarily due to ligand exchange reactions
where hydroxide ions replace phosphate. Calcite and apatite formation
at higher pH-values increase the P-binding capacity of calcium |
Temperature | An increase in temperature gives primarily
indirect effects due to increased bacterial activity, which increases
oxygen consumption and decreases the redox potential and pH-value. The
production of phosphate-mobilizing enzymes and chelating agents might
increase accordingly |
Equilibrium criteria | Affects adsorption-desorption and dissociation of precipitates
|
Chelating agents | Replace phosphate from salts
with calcium, iron and aluminum. Chelating agents can be produced by
bacteria and algae or occur as a pollutant |
Soil P status: Soil chemistry and Soil biology
Theoretical examples of how soil factors may affect risk of P transfer (Haygarth, 1997)
Factor | Effect | Risk |
High P status | large pool of PTP (potentially transferable P) | high |
High Ca, Al or Fe status | Precipitation of P, reduced chance of transfer to soil solution | low |
Waterlogged- reducing conditions | P precipitates with Fe and the precipitate is mobilised to solution | high |
High organic matter status | P immobilised | low |
Lime added increased pH | Above pH 6, P sorbed to Fe and Al oxides Below pH 5, P sorbed to Fe and Fe oxides butNB. Effect of pH is not easily simplified | low high (pH range 5-6) |
High clay content | Poor vertical drainage, high chance of surface runoff High P sorptive capacity | high low |
Humic and Non-humic lakes in Atlantic Canada
(Janus and Vollenweider, 1981)
Non-humic lakes in the Atlantic
Region seem to conform with the expected lake behavioural pattern
established by the (nearly 150) OECD lakes. Other lakes in the Atlantic
Region exhibit particular properties, e.g. high colour caused by humic
substances, which cause them to depart from the standard behavioural
pattern derived from the OECD lakes. In these coloured lakes, a
substantial part of the total phosphorus present is associated with
dissolved organic substances and is not readily available for
phytoplankton growth. For this reason, humic, coloured Atlantic Region
lakes at a given phosphorus concentration maintain a lower algal
biomass as indicated by chlorophyll, than that predicted from the OECD
relationship.
Phosphorus associated with
dissolved humic substances is not eliminated from a lake as readily as
phosphorus associated with phytoplankton. Flushing corrected
predictions underestimate lake concentrations of total phosphorus, but
this compensates somewhat for the less mobile nature of phosphorus in
humic lakes.
Therefore, average
chlorophyll values predicted from loading fall below but nearer the
OECD relationship than those predicted from measured lake phosphorus
concentration. Peak chlorophyll is even more closely predicted from
loading than average values. This is a consequence of the equalization
of the compensating effects. The proportion of total phosphorus which
is available for plant growth may increase at times of peak biomass and
reflect a finite binding capacity for inactivation of phosphorus.
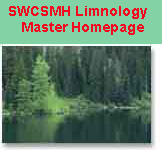
We salute the Chebucto Community Net (CCN) of Halifax, Nova Scotia, Canada for hosting our web site, and we applaud its volunteers for their devotion in making `CCN' the best community net in the world!